28 de August de 2024
Sport tech and science Velocity Based Training
High loads vs low loads adaptations
It is well known that when implementing velocity-based training maximum intention must be applied in every repetition. In this sense, maximum force can be improved with any load when the maximum intention is achieved. However, not all loads create the same adaptations. Similarly, not every exercise in some circumstances allows the athlete to achieve the maximum output. Therefore, in this article, I am going to speak about the physiological adaptations taking place when using different loads (high vs low) and which kind of exercise benefits more in each case.
Well, when we are developing the athlete, it is clear that the athlete does not have much time in sport-specific actions to apply the maximum force. Maximum force is usually achieved around 200-300 ms after the onset of the contraction, while sport-specific actions usually last for less (80 to 250 ms). Even though time to produce force is limited, the improvement in the velocity with high loads will be of help to improve the velocity of lighter loads. Indeed, when improving the velocity with higher loads, lighter loads will represent a lower relative intensity for the athlete. That is why the absolute levels of force have been directly linked to performance in sport-specific actions such as sprinting, change of direction, and jumping ability.
We all know that barbell maximum velocity is a must in all lifts to enhance neuromuscular adaptations. Also, different velocities are expected with different loads (i.e., slower with heavy loads and faster with lighter loads). However, behind barbell velocity, it is extremely important to know which physiological adaptations are taking place in each case. So, in the next part of the article, we will focus on the physiological adaptations taking part with heavy and moderate-light loads.
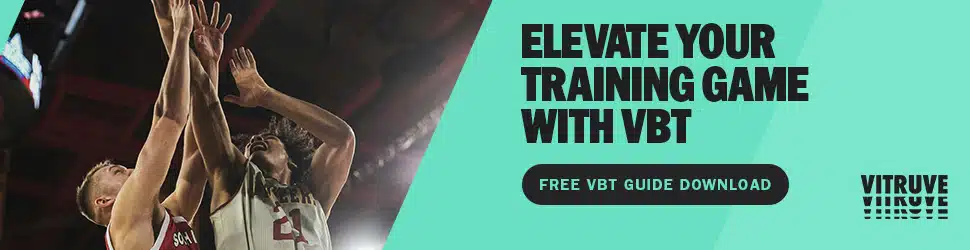
Motor unit recruitment:
To start explaining this, we must understand that the magnitude of muscle activation and hence the amount of force produced depends on the number of motor neurons activated (MU recruitment) and the frequency at which MUs discharge action potentials (discharge rate). These two physiological mechanisms are maximized differently and are dependent on the time. As Maffiuleti et al. stated, the relative contributions of recruitment and discharge rate modulation to the force exerted by a muscle vary with contraction speed. This can be partially explained by the law of size that the MU recruitment follows. The law of size states that in order to recruit the high threshold motor units, low threshold have to be recruited before. Therefore, slow contractions are characterized by a progressive activation of MUs. However, the reduction in MU activation is more marked for slow-contracting muscles (e.g., soleus) than fast-contracting (e.g., masseter). This does not mean that you have to lift slow to maximize MU recruitment, but that higher loads lifted at maximum velocity that are actually associated with slower speed will maximize MU recruitment. Curiously, traditional resistance exercises have perfect characteristics for this, and we will speak about this in the next part of the article. To illustrate this a little bit more, you can take a look at figure one and realize that the maximal voluntary contraction, which is highly dependent on MU recruitment, is greatly associated with the rate of force development at the latest stages of the contraction.
Motor unit discharge rate:
On the other hand, discharge frequency is also of extreme importance. MU recruitment is only able to achieve 80-90% of the maximal voluntary contraction; the increase of maximal voluntary contraction beyond this is entirely due to an increase in discharge rate. Discharge rate triggers an amplified magnitude of Ca2+ efflux to the cell cytosol, evoking a high rise in the contractile force development. In this case, fast contractions are incredibly important. Indeed, rapid contractions are characterized by a high initial discharge rate at the onset of activation that declines progressively with successive discharges. Contrarily to MU recruitment, discharge frequency can reach maximum values instantaneously. Studies have reported that MU discharge frequency can reach instantaneous values between 60-120 Hz at the onset of a rapid contraction but a maximum frequency between 30-60 Hz in a high force isometric contraction. As Maffiuleti stated in his study in 2016, “the ability to produce force rapidly depends predominantly on the increase of muscle activation at the onset of the contraction and less on the speed-related properties of the muscle.” For all of this, we can state that discharge frequency is of more importance to increase the maximal voluntary contractions in fast actions due to the inability to recruit high threshold MUs. However, we have to account for several factors besides discharge frequency for the increase in the early RFD. The early RFD also depends on muscle contractile properties, maximal voluntary contraction, and neural drive.
Summarizing the above, MU recruitment and discharge rates follow different patterns. While MU recruitment is highly associated with the RFD in late stages of the contraction, MU discharge rate is greatly associated during the onset.
- MU recruitment would help to increase the maximal voluntary contraction which accounts for the 80% in the variance of the RFD during the later phase.
- Discharge rates would be of more influence during the early phase. However, discharge rate would only explain the variance in the early RFD up to a 35% being the contractile properties of the muscle of more importance.
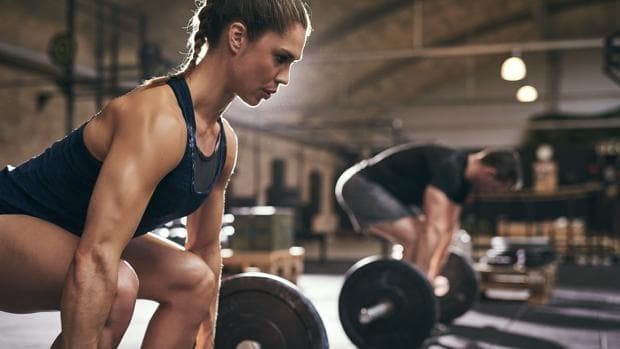
RFD (Rate of Force Development) Curve and Velocity-Based Training
Adaptations with traditional resistance exercises
Now that we have understood the physiological mechanisms taking place with slow vs fast contractions, I am going to present the different exercises that I would recommend for each. Typically, and in my opinion, traditional resistance exercises are more suitable for training with high loads due to the biomechanical reasons I will speak about. Contrarily, ballistic exercises allow for rapid contractions and are more suitable for moderate-light loads.
When trying to optimize training, exercise selection is of incredible importance. In this paragraph, I will speak about traditional resistance exercises. Traditional resistance exercises are importantly characterized by a braking phase taking place at the end portion of the lift in which the athlete is applying force in the opposite direction to the load’s motion when moderate to light loads are used. Therefore, maximum force production is limited with moderate to light loads in this type of lift. Let’s divide the lift into two portions: propulsive and braking. The onset of the braking phase is placed when acceleration reaches values below -9.91 m/s². This braking phase is importantly increasing with lighter loads and slightly insignificant with loads above 70%. To our knowledge, from 76% the contribution is entirely propulsive, highlighting the importance of the use of high loads to attain the maximum output in traditional resistance exercises. For this reason, accommodate resistance exercises have been proposed as a good alternative to continuously maintain the acceleration of the barbell until the end of the lift. However, I would consider those exercises faster than traditional resistance exercises as the load to use would expect the braking phase to be significant (below 76%) and they also reduce the mechanical work performed around the sticking point where, in my opinion, the most interesting part of the lift is performed. So, I would use those variations of resistance training exercises close to competition or during a peaking phase where we want to limit the fatigue and the amount of work performed at slow velocities. Contrarily, I would use resistance training exercises when trying to develop the absolute strength of an athlete.
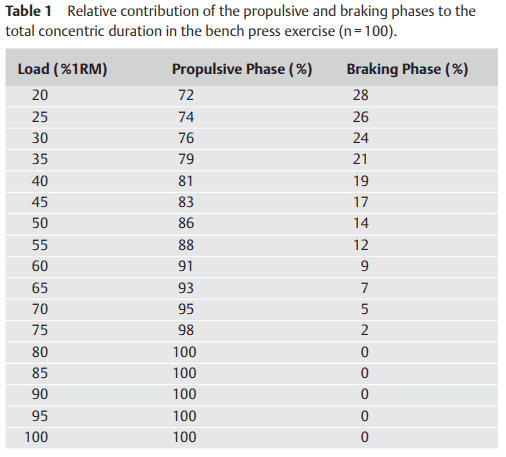
Importantly, the output of resistance training exercises with lighter loads may be limited when trying to apply the maximum force as they encourage the athlete to jump. Indeed, when calculating the load-velocity relationship, loads above 40-45% are recommended if traditional resistance exercises are used. On the other hand, these exercises usually exert slower velocities and possess longer durations than ballistic exercises, allowing the athlete to achieve greater force outputs due to MU recruitment, especially with high loads.
Another important aspect of traditional resistance exercises is the high mechanical stress and time under tension. It is well known that a really important aspect to increase the rate of force development is the muscle cross-sectional area. Then, if I would have to use any exercise to develop muscle mass, especially during a general preparation part or a block where we are looking to develop the athlete’s structure, I would choose without a doubt traditional resistance exercise. Especially in compound exercises, the mechanical tension can be maximized due to the greater capacity to mobilize greater loads. Regardless of the metabolic stress, which actually is an indirect mechanism that increases mechanical tension in the muscle cell due to muscle swelling, mechanical tension has been proved to be one of the most important mechanisms to activate MTORC1, one of the most important pathways to activate protein synthesis. Also, the greater time under tension in comparison with ballistic or explosive exercises allows for greater hypertrophy results. Obviously, muscle mass increases are of importance for athletes. Despite the fact that it must appear as an indirect consequence of training in most sports, in some cases (rugby and American football) will be directly developed by coaches.
In the last case, I would like to specify that for long-term development, the increase of the cross-sectional area is incredibly important. Especially in the USA, where high schools and colleges have the opportunity to keep the athletes for several years. It is obvious that in a context of athletic development, greater velocity losses may not always be adequate. However, I would like to state that in most of the studies that a lower velocity loss has reported greater results in sport-specific abilities are short-term and those differences may have taken place due to an increased readiness or a “peaking” effect in the group of lower velocity loss. It is yet to be clarified if the velocity losses reported in those studies can generate improvement in the long term.
The curiosity:
In a previous post, we have been treating the topic of mean propulsive velocity vs peak velocity to track the speed of the barbell. Well, this braking phase is the main reason why mean propulsive velocity is recommended for traditional resistance exercises. Mean propulsive velocity only accounts for the velocity attained in the propulsive phase and eliminates the one attained in the braking. On the other hand, mean velocity does actually account for the velocity of the braking phase. This is the reason why in some studies mean velocity has been proven not to be sensitive enough to detect changes in performance. Then, in case you are tracking the velocity of loads under 75% of the 1RM with the mean velocity, you should know that the braking phase will be influencing the result. My recommendation is to always use the mean propulsive velocity regardless of the load for this type of exercise.
Adaptations with ballistic exercises:
Contrary to resistance training exercises, ballistic exercises do not possess the braking phase as the athlete is continuously accelerating the barbell through the lift. The elimination of the braking phase and the continuous acceleration in the lift allow the athlete to achieve greater velocities in shorter contraction times. Therefore, don’t limit the output with light loads and use them with the correct type of exercises. In the image below, we can observe how the peak force attained with the same relative intensity in a ballistic exercise is greater in comparison with the non-ballistic variation. Not only that, but the slope of net force is more inclined, indicating a greater rate of force development (RFD) in that exercise.
Lastly, I would like to write a little bit about specificity, especially about contraction times. Usually, to get an optimal outcome in both exercises, different contraction times are required. Ballistic exercises usually achieve greater velocities, making them closer to sport actions. Then, based on kinematical demands, those kinds of exercises would be more sport-specific than traditional exercises.
MU discharge rate has been proved to increase with ballistic type exercises (Van Cutsem et al. 1998). Indeed, explosive and ballistic exercises can maximize very large increases in the discharge frequency at the onset of the contraction, along with an increased ability to sustain a high discharge rate from the first to the third interspike interval. Discharge rates are 2-3 times higher during ballistic than slow contractions. Additionally, ballistic exercises have been reported to increase the incidence of doublet discharges (i.e., successive MU action potentials with an interspike interval <5 ms), which is known to result in an increase in contractile force production and larger increases in RFD. These doublet discharges can increase the order of 5% to 33% of all MUs recorded after a 12-week period of ballistic training. Additionally, ballistic contractions have been shown to decrease the recruitment threshold due to a greater RFD demand.
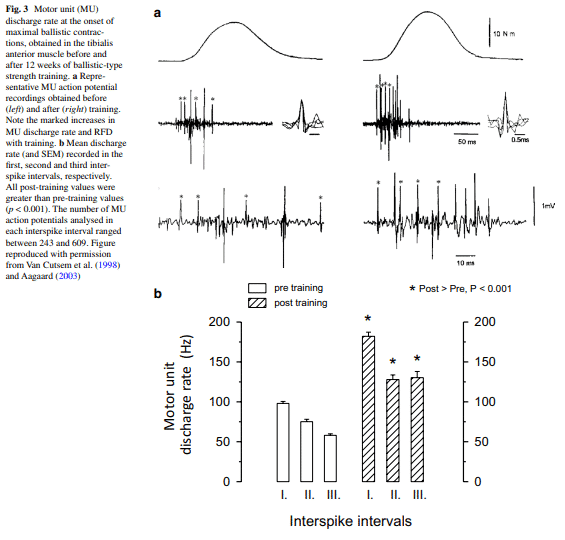
To conclude:
In conclusion, use each type of exercises and loads wisely. Velocity-based training allows coaches to optimize training and should help them to make decisions. Velocity-based training is not a training method in which you should attain the highest velocity with light loads. Each kind of load produces specific adaptations and the continuous use of ridiculously light loads in traditional resistance exercises with no intensity whatsoever won’t help your athlete to achieve its greatest potential. So, I hope this article can help you to understand why you should select the right type of exercise with the right type of load in any given context you might be in.
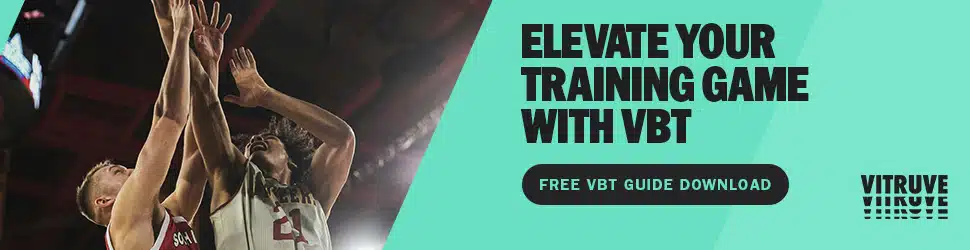
References:
1. Enoka, F. G., & Stuart, M. D. (1992). The rate of force development of human skeletal muscle.
Journal of Applied Physiology, 72(5), 1637-1648. doi:10.1152/jappl.1992.72.5.1637
2. Maffiuletti NA, Aagaard P, Blazevich AJ, Folland J, Tillin N, Duchateau J. Rate of force
development: physiological and methodological considerations. Eur J Appl Physiol. 2016
Jun;116(6):1091-116. doi: 10.1007/s00421-016-3346-6.
3. Duchateau J, Enoka RM. Human motor unit recordings: origins and insight into the integrated
motor system. Brain Res. 2011; 1409:42–61
4. De Luca CJ, LeFever RS, McCue MP, Xenakis AP. Behaviour of human motor units in different
muscles during linearly varying contractions. J Physiol. 1982; 329:113–128
5. Kukulka CG, Clamann HP.Comparison of the recruitment and discharge properties of motor units
in human brachial biceps and adductor pollicis during isometric contractions. Brain Res. 1981;
219:45–55
6. Desmedt JE, Godaux E. Ballistic contractions in fast or slow human muscles: discharge patterns
of single motor units. J Physiol. 1978; 285:185–196
7. Duchateau J, Hainaut K. Nonlinear summation of contractions in striated muscle. II. Potentiation
of intracellular Ca2+ movements in single barnacle muscle fibres. J Muscle Res Cell Motil. 1986;
7:18–24
8. Binder-Macleod S, Kesar T. Catchlike property of skeletal muscle: recent findings and clinical
implications. Muscle Nerve. 2005; 31:681–693
9. Desmedt JE, Godaux E. Ballistic contractions in man: characteristic recruitment pattern of single
motor units of the tibialis anterior muscle. J Physiol. 1977; 264:673–693
10. Klass M, Baudry S, Duchateau J (2008) Age-related decline in rate of torque development is
accompanied by lower maximal motor unit discharge frequency during fast contractions. J Appl
Physiol. 1985; 104:739–746
11. Van Cutsem M, Duchateau J. Preceding muscle activity influences motor unit discharge and rate
of torque development during ballistic contractions in humans. J Physiol. 2005; 562:635–644
12. Van Cutsem M, Duchateau J, Hainaut K. Changes in single motor unit behaviour contribute to
the increase in contraction speed after dynamic training in humans. J Physiol. 1998; 513(Pt
1):295–305
13. Andersen LL, Aagaard P. Influence of maximal muscle strength and intrinsic muscle contractile
properties on contractile rate of force development. Eur J Appl Physiol. 2006 Jan;96(1):46-52.
doi: 10.1007/s00421-005-0070-z.
14. Sanchez-Medina L, Perez CE, Gonzalez-Badillo JJ. Importance of the propulsive phase in strength
assessment. Int J of sports med. 2010; 31(2), 123–129. https://doi.org/10.1055/s-0029-1242815
15. Sánchez-Medina L, Pallares JG, Pérez CE, Morán-Navarro R, González-Badillo JJ. Estimation of
relative load from bar velocity in the full back squat exercise. Sports med int open. 2017; 1 :E80-
E88. Doi :10.1055/s-0043-102933
16. Lake J, Lauder M, Smith N, Shorter K. A comparison of ballistic and nonballistic lower-body
resistance exercise and the methods used to identify their positive lifting phases. J Appl
Biomech. 2012 Aug;28(4):431-7. doi: 10.1123/jab.28.4.431.